What are ice wedges, and why are they important to climate change?
An in-depth look at this unique feature of the Arctic landscape
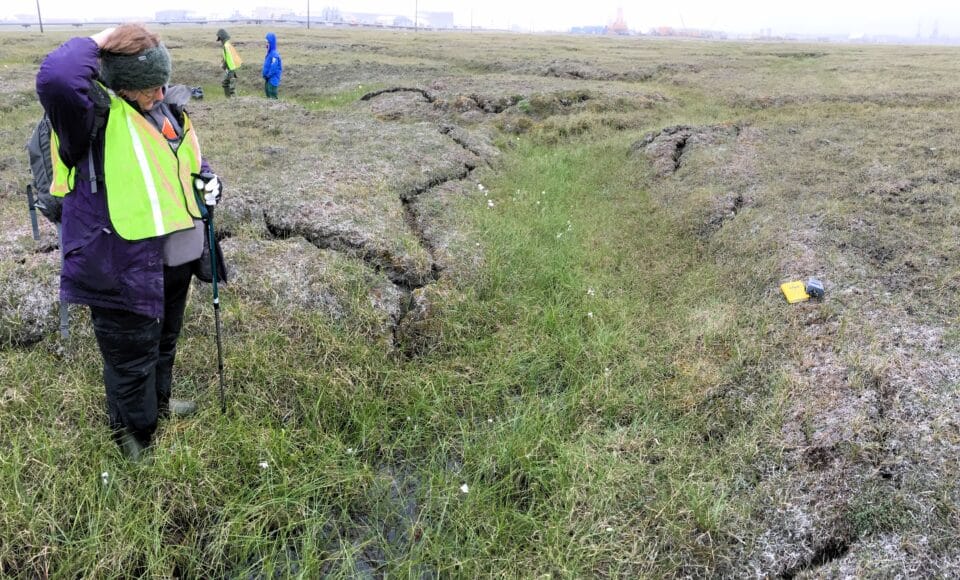
Ice-wedge polygons on the surface of the Arctic tundra in Prudhoe Bay, Alaska.
photo by Anna Liljedahl
“I think ice wedges are what make permafrost interesting,” says Dr. Anna Liljedahl.
Liljedahl works on Woodwell Climate’s Arctic team as an Associate Scientist. She aims to understand how climate change is affecting water storage and movement. Much of her recent work focuses on ice wedges and how they are reacting to warming Arctic summers. But just what are ice wedges anyway?
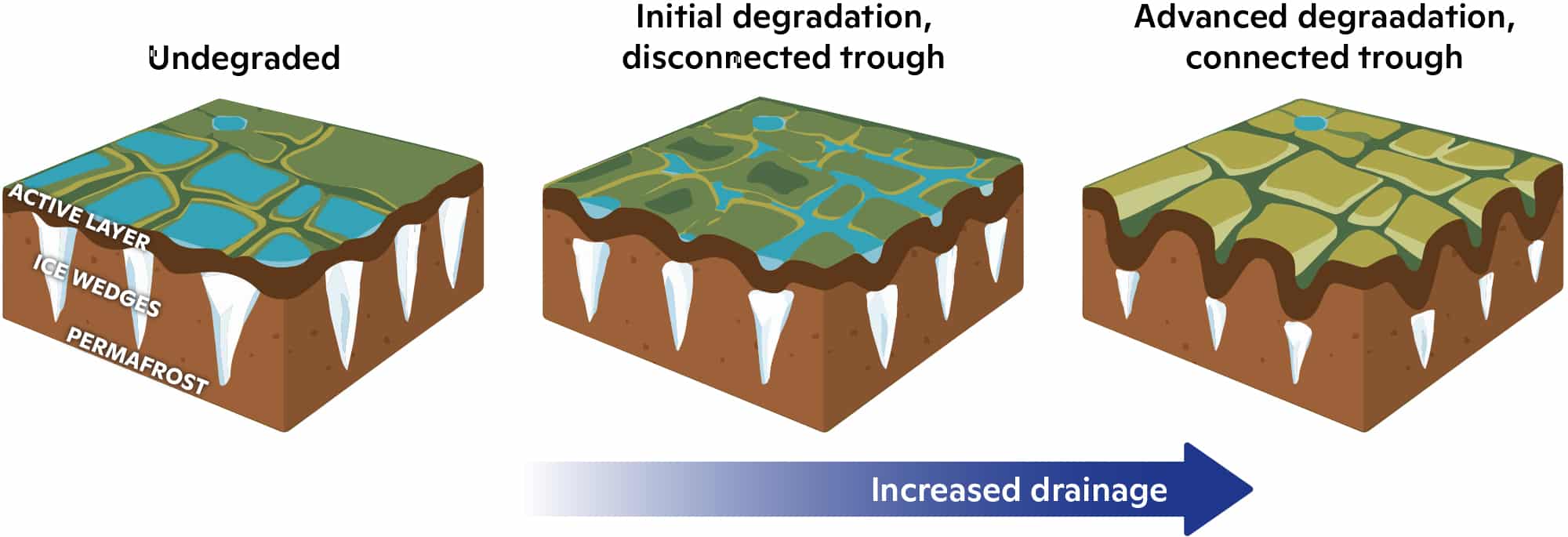
Graphic demonstrating ice-wedge degradation from Liljedahl et. al’s 2016 article “Pan-Arctic ice-wedge degradation in warming permafrost and its influence on tundra hydrology” published in Nature Geoscience Journal.
figure courtesy of Anna Liljedahl, with minor design edits by Julianne Waite
Ice wedges are one of the three main features of the Arctic’s land surface. Permafrost, ground that remains below 0˚C for at least two consecutive summers, lies under a thinner layer of thawing and refreezing soil, called the active layer. When permafrost cracks during cold winter days, snowmelt and runoff water seep into the empty space. These eventually freeze and create a wedge-shaped spear of ice that extends vertically down into the permafrost.
Ice wedges actively re-shape the tundra. When they freeze, they grow and expand outward, pushing against the bordering permafrost and active layer. With nowhere else to go, permafrost and soil push upwards, and ridges form on the surface of the tundra. The ridges interlock and form distinct shapes, referred to as ice-wedge polygons.
The ridged borders of ice-wedge polygons form directly above expanding ice wedges below the surface, and are therefore more elevated. The lower internal portion of the polygon allows pools of water from runoff and snowmelt to form atop the active layer. These polygons are visible all the way from space.
Thanks to satellite imagery, scientists like Liljedahl are able to monitor ice-wedge polygons remotely. Satellite images date back to the mid-20th century and can be used to observe changes in the landscape overtime.
During unusually warm summers, the tops of ice wedges can melt, which removes underlying support of the ground surface, causing slumps along the borders of ice-wedge polygons. These leveling borders form channels that siphon the water from pools in the centers of neighboring polygons. The resulting runoff streams can drain small pools and even larger lakes that took thousands of years to form.
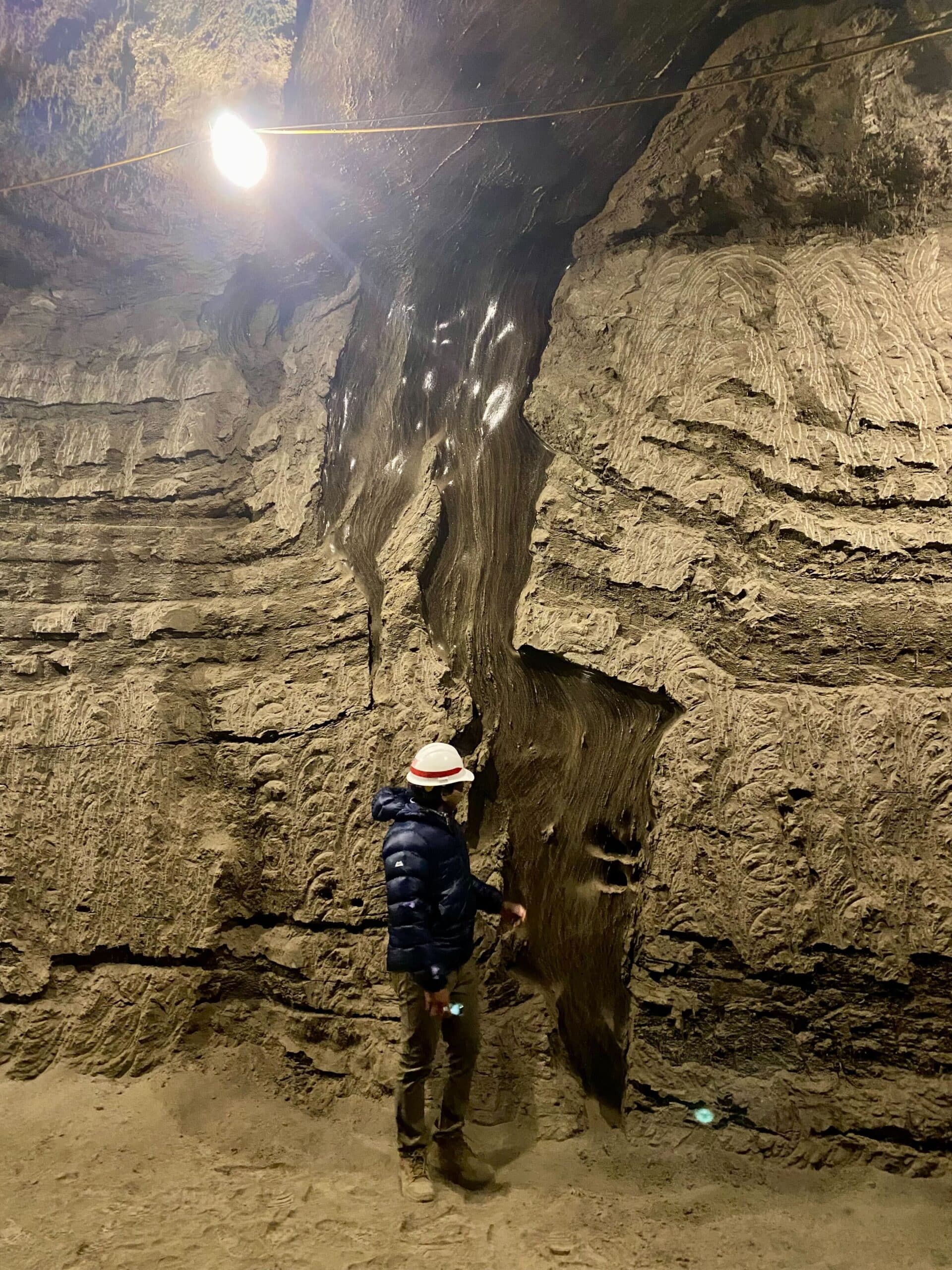
An ice-wedge striking through permafrost at the Cold Regions Research and Engineering Laboratory (CRREL) Permafrost Tunnel in Fairbanks, Alaska.
photo by Anna Liljedahl
With the progression of climate change, these drainage systems have become more common. Liljedahl refers to them in the title of her manuscript, just published in the July issue of Nature Water, “The Capillaries of the Arctic Tundra.”
The increase in creation of new “capillaries” in the Arctic is impacting not only the topographical landscape of the region, but also the livelihoods of all beings that find their home there.
At first, the melt of these ice wedges can spark an uptick in the variation of vegetation due to moisture along the sides of the channel. This, however, is temporary. When the ice wedges stabilize again in the winter, this variation decreases once more.
Aquatic mosses—one of the most productive vegetal forms in the Arctic, equivalent in productivity to Arctic shrubs—inhabit pools formed alongside the edges of ice-wedge polygons. They lose their homes when bodies of water drain away. Major vegetation changes can alter carbon storage, availability, and emissions across the tundra.
Humans are also impacted. Homes become too dangerous to live in as the ground supporting vital infrastructure collapses. Roads connecting communities to important resources are destroyed by subsiding ground.
Despite their widespread impact, ice wedges are often overlooked in Arctic climate models. Historically, their inclusion “costs too much computer time,” Liljedahl says, to factor in. Many climate models take a holistic approach to the Arctic landscape, as opposed to focusing on smaller details.
To remedy this, Liljedahl suggests utilization of developing technology such as Artificial Intelligence (AI). Classifying the Arctic landscape by type, for example, into high-center polygons, low-center polygons, and capillary networks, would factor ice wedge change into climate models. As AI advances and becomes a more common research tool, it could help decrease the human computing time that Liljedahl identifies as a barrier.
Arctic research is likely to change drastically in the coming years. With new technologies, and as we learn more about the Arctic landscape, research models will likely become more inclusive of the varied features within it, and much more accurate.
“There are exciting years ahead,” Liljedahl says, “I think we’re going to see some cool stuff coming out [of tundra research] in the next five to ten years.”